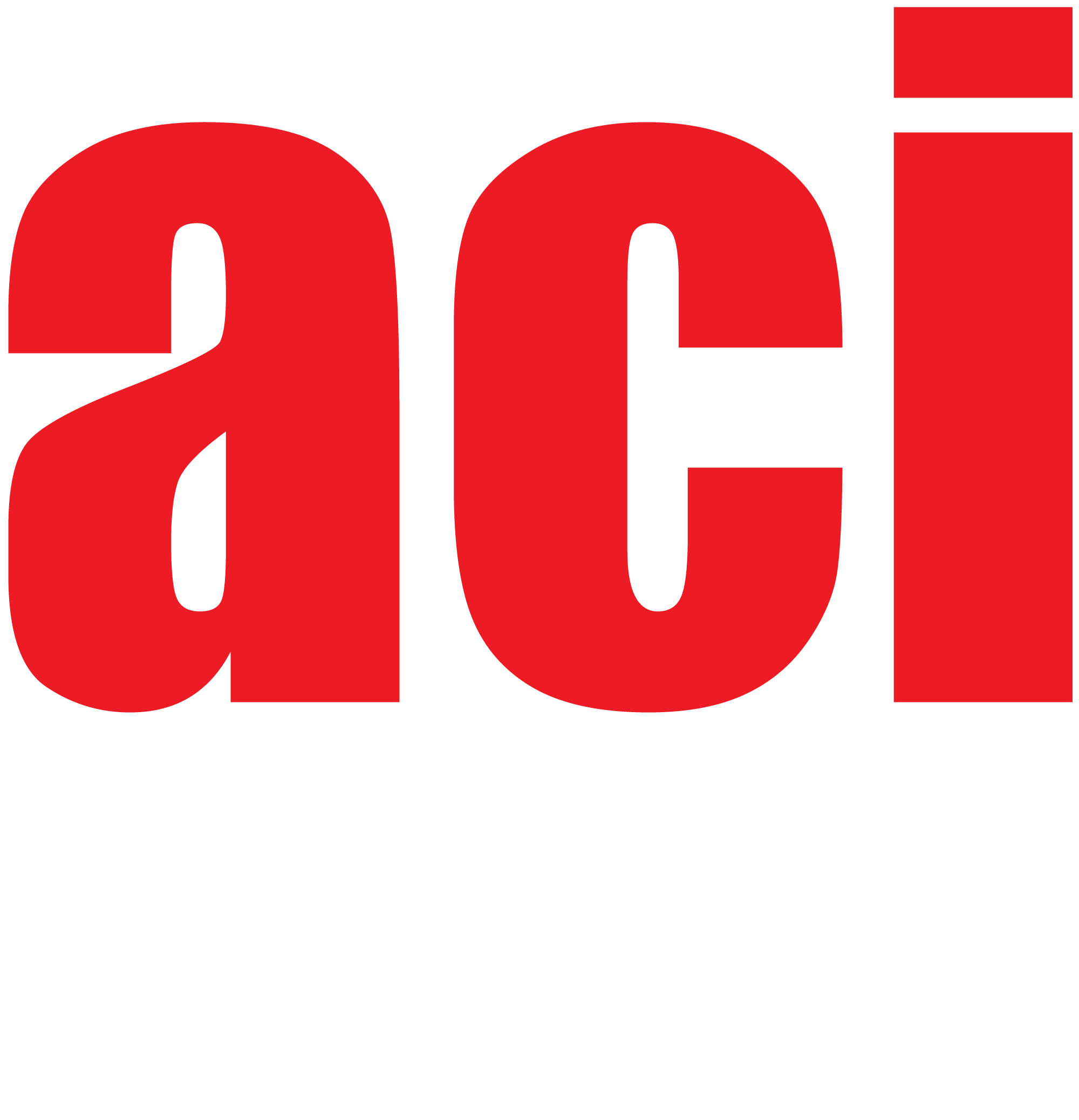
SECCIÓN B: CIENCIAS DE LA VIDA
La bacteria oportunista Pseudomonas aeruginosa es una de las principales preocupaciones como agente etiológico de infecciones nosocomiales en humanos. Muchos de los factores de virulencia utilizados para colonizar el cuerpo humano son los mismos que utiliza P. aeruginosa para prosperar en el medio ambiente, como el transporte por membrana, la formación de biopelículas y la reacción de oxidación/reducción, entre otros. El origen de P. aeruginosa es principalmente el medio ambiente, la adaptación a los tejidos de los mamíferos puede seguir un modelo de evolución fuente-sumidero; el medio ambiente es la fuente de muchos linajes, algunos de ellos capaces de adaptarse al cuerpo humano. Algunos linajes pueden adaptarse a los humanos y pasar por una evolución reductora en la que se pierden algunos genes. La comprensión de este proceso puede ser fundamental para aplicar mejores métodos de control de brotes en los hospitales.
viewed = 444 times