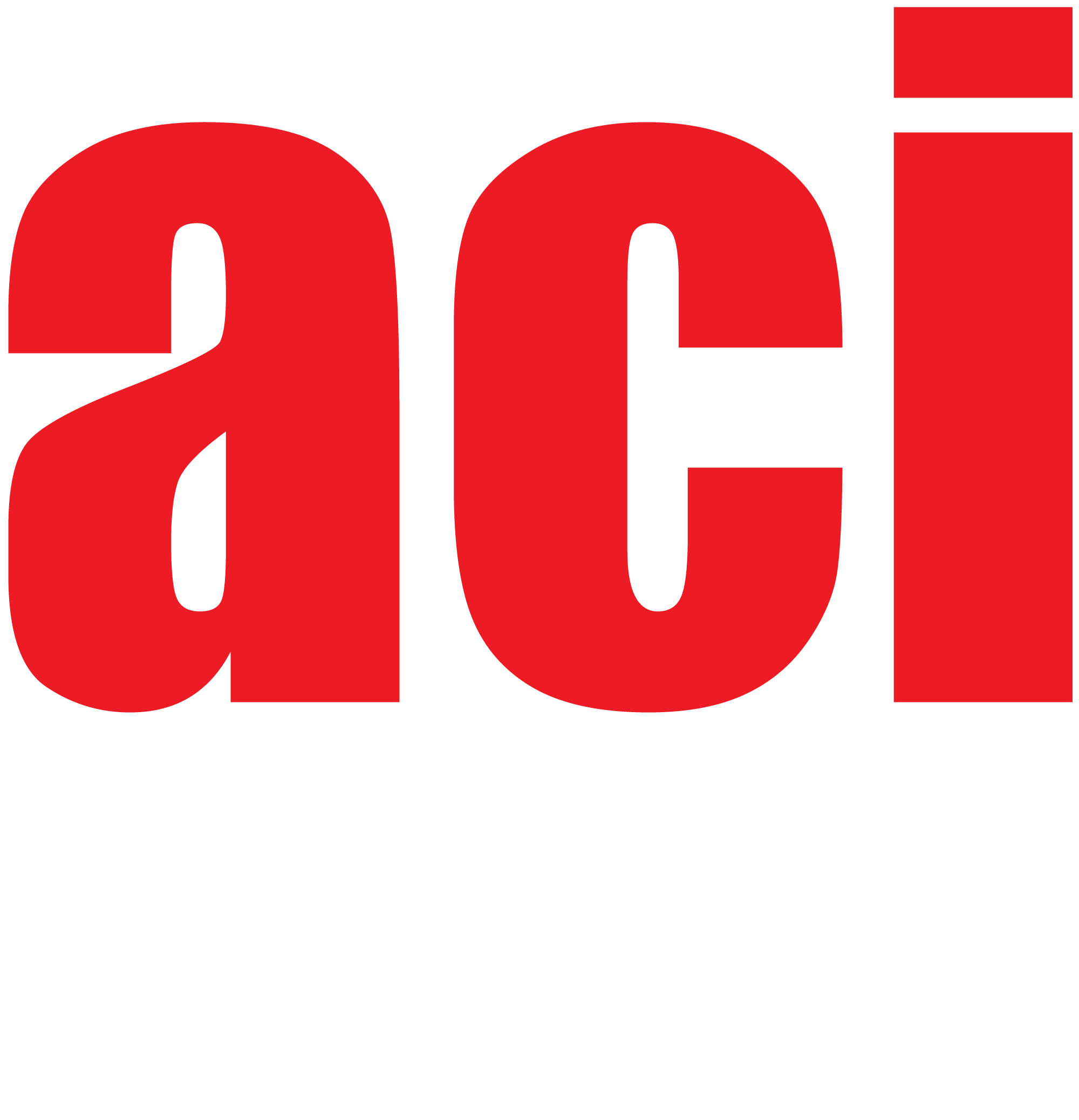
SECCIÓN A: CIENCIAS EXACTAS
A data driven solution to the dark matter problem is presented. This short and self-contained overview is intended for a wide audience, with full technical details available in the cited references. We present redundant, independent and consistent measurements of the dark matter particle comoving root-mean-square velocity vhrms(1), or equivalently, of the dark matter temperature-to-mass ratio. These measurements agree with the “no freeze-in and no freeze-out” scenario of spin zero dark matter that decouples early on from the Standard Model sector, e.g. spin zero dark matter coupled to the Higgs boson or to the top quark.
About this paper
The present article was published on April 13th, 2023 in the European Journal of Applied Sciences and it has been assigned a DOI by the EJAS. The work is republished by ACI Avances en Ciencias e Ingenierías according to the creative commons license (Attribution 4.0 International, CC BY 4.0) used by the EJAS, and according to the copyright preserved by the author, Bruce Hoeneisen. Readers can access the original publication via the following link: https://journals.scholarpublishing.org/index.php/AIVP/article/view/14383 or through the DOI: https://doi.org/10.14738/aivp.112.14383.