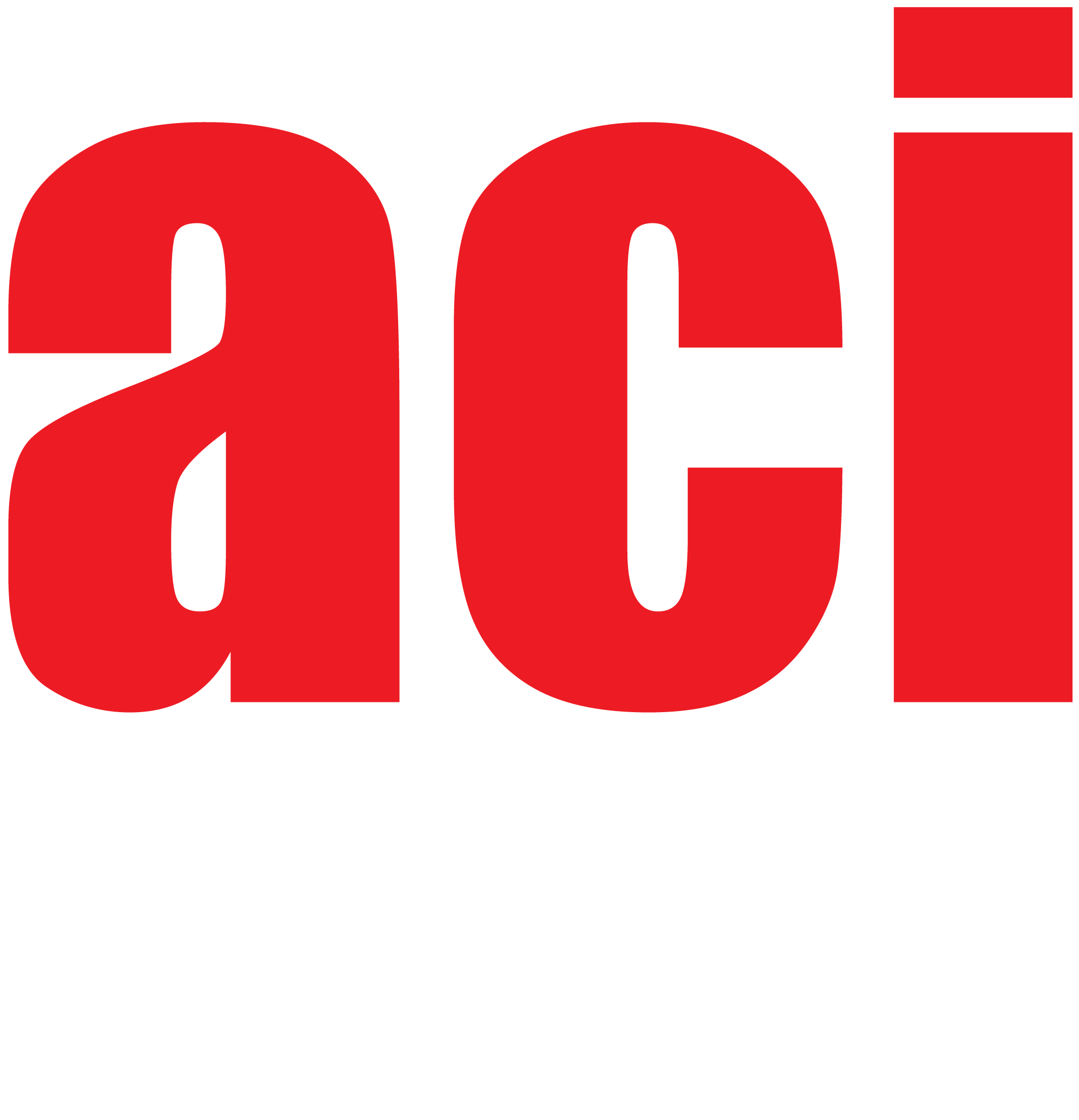
SECTION C: ENGINEERING
Liquefied petroleum gas (LPG) is essential, mainly for domestic use, representing 10.4% of Ecuador's energy demand. In the absence of mechanisms for the valorization of lignocellulosic waste in the country, a biomass-to-butane conversion route was proposed as a substitute for LPG through a computational design to evaluate its technical, economic and environmental feasibility. The optimal waste for the process was selected based on the annual generation rate and physical-chemical composition. The route configuration was designed in AspenPlus® with an input of 77 t/h biomass. Based on the results, an economic and life cycle analysis was carried out using the openLCA® software. Butane production reached seven tons per hour and an energy density of 26.7 MJ/L.
Regarding the economic axis, the minimum sale price calculated was $1.03/kg, considering the sale of lignin as a co-product. In this way, the biofuel was competitive with the sale price of one dollar per kilogram of LPG. Finally, the total carbon footprint of the process was 102 g CO2-eq/MJ, higher than the European standard of 94 g CO2-eq/MJ. This research opens the door towards optimizing resources and transforming the country's energy matrix.
viewed = 515 times